August 10, 2006 feature
Detector can count atom by atom
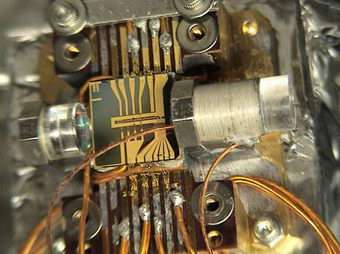
More than 80 years have passed since Louis de Broglie discovered that matter can act like a wave as well as a particle. With advances in technology, scientists have recently begun exploiting this strange property of everyday matter for applications such as atom lasers and nanofabrication. To further their understanding of these applications with matter waves, however, scientists need a way to detect and count the exact number of atoms used.
MIT scientists Igor Teper, Yu-Ju Lin and Vladan Vuletic have developed a method for counting individual atoms that will likely assist in future developments of many different types of atom optics devices. Using an optical cavity – an arrangement of mirrors that forms a standing wave (a major component of lasers) – the detector counts atoms by detecting the photons that scatter off the atoms, either into the cavity or out of it. The group’s results are published in a recent issue of Physical Review Letters.
In atom optics devices, atoms must be cooled to temperatures close to absolute zero (-459 degrees Fahrenheit) in order for them to exhibit their wave-like nature. Such ultra-cold atoms can then be manipulated much like light waves, but fully exploiting this ability requires high atom-number resolution.
“Many interesting and novel atom optics applications, such as quantum information processing, that could potentially be implemented on atom chips require precise knowledge of atom numbers and atom statistics, and, in some cases, the ability to prepare and detect single atoms,” Teper told PhysOrg.com. “The challenge is that the signal from a single atom is generally very small, so you need to either collect the signal for a long time or find some way to enhance the signal and reduce background noise. In our case, the cavity does both, since it enhances atom scattering and also filters out most of the background light.”
In their set-up, Teper, Lin and Vuletic first trapped and cooled rubidium atoms in a magnetic trap. The team used two different measurements – fluorescence and absorption – to detect the photons scattering off the atoms. The fluorescence method works by illuminating the atoms with laser light of a specific frequency and measuring the amount of laser light scattered into the cavity. Although with this method atom number resolution decreases with larger quantities of atoms because larger light signals have larger fundamental uncertainty, the method allowed detection of a single atom with 75% efficiency in 250 microseconds.
“There are various limits on how long ultracold atom experiments can take, both in terms of how long the atoms survive in the trap before they are lost and also in terms of how long various properties of the atoms are preserved,” said Teper. “One would want atom number measurements to take much less time than these limits so that one can perform the detection, or possibly many detections, without significantly cutting into the time available for the rest of the experiment. Also, one may need to measure an atom in motion, so that it spends only a short time in the detection region. Different applications can have very different constraints on the detection time.”
Using the absorption method, the scientists measured the rate of photons taken in by an atom, in effect looking at the atoms’ shadow, and achieved a resolution of about one atom. Because more atoms absorb more photons, which reduces the observed light signal, the absorption method is not limited the way fluorescence is when measuring a large number of atoms. Using a combination of fluorescence and absorption methods provides versatility for counting atoms in different applications.
“Current atom optics capabilities include atom lasers, mirrors and beam-splitters for matter waves, atom interferometry (using both thermal atoms and Bose-Einstein condensates (BECs)), and BEC Josephson junctions,” said Teper. “Some of these have been achieved in magnetic traps on chips, some in free-space magnetic traps, some in optical traps, and some with untrapped atoms.”
Many atom optics applications seem very futuristic, but the laws of quantum mechanics also seem to predict that such applications are possible with the proper technology. For example, scientists speculate on atom holography, which would be like laser holography but would replicate objects with actual matter instead of light. Also, more advanced atom lasers might act like the opposite of an optical laser, using light to direct beams of matter waves.
“There has been rapid progress in the past few years in all these experimental regimes; many technical problems have been solved, and there's a lot of excitement in the field,” said Teper. “BEC interferometry with magnetically trapped atoms on chips is one area where there's been a series of breakthroughs recently, and there's lots of interesting experiments ahead. Quantum information processing is one of the big goals of atom optics on chips, and while there's been a lot of progress on some of the necessary components, there's still a tremendous amount of work to do and many challenges to overcome.”
Citation: Teper, Igor, Lin, Yu-Ju and Vuletic, Vladan. ”Resonator-Aided Single-Atom Detection on a Microfabricated Chip.” Physical Review Letters 97 023002 (2006).
By Lisa Zyga, Copyright 2006 PhysOrg.com